During the period 2018 – 2022, LUMES researchers have conducted their activities within three main research areas: ultrafast laser science, laser-matter interaction, and quantum materials science. The research has been organized into 10 distinct research lines, comprising eight well-established lines with a consistent scientific output over time (a minimum of 10 publications in JCR) and two emerging lines that initiated their scientific activities during this reporting period
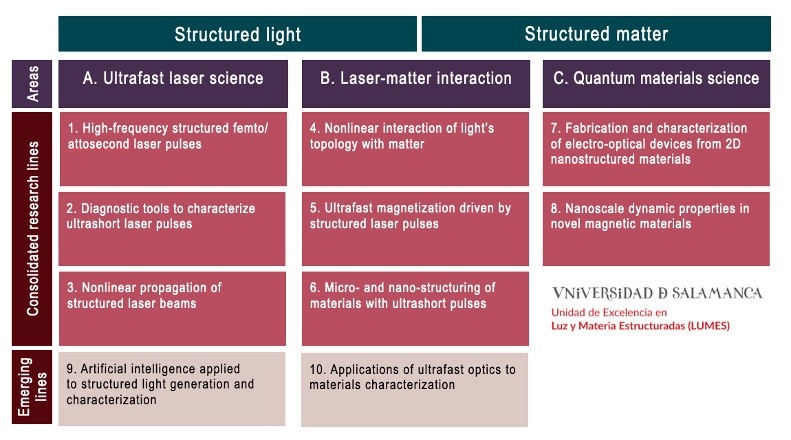
Details of LUMES research lines
1. Generation of high-frequency structured laser pulses in the femto and attosecond timescales
Participating researchers | 4 Principal investigators, 6 senior investigators, 3 PhD students |
Publications related | 46 publications Nature Photonics 12, 349 (2018), Science 364 (2019), Nature Photonics 13, 123 (2019), Science Advances 7, (2021), Optica 9, 71 (2022), Science Advances 8, (2022) |
Projects related | EU (ATTOSTRUCTURA ERC2019), National (PID2019-106910GB-I00, PID2022-142340NB-I00, FIS2016-75652-P), Beca Leonardo BBVA 2017 |
Theses | One defended, two in progress |
During the last few years, high-frequency laser radiation has emerged as the only tool capable of enabling the observation and manipulation of nature on both the shortest time scales (attoseconds) and the smallest spatial scales (nanometers). The University of Salamanca (USAL) has been a pioneer in the development of structured high-frequency laser light sources with unique properties in the temporal, spatial, and polarization domains. Since 2009, USAL has been engaged in theoretical simulations at the forefront of knowledge in theoretical modeling. These simulations provided support for two pioneering experiments conducted by the research group led by Profs. Henry Kapteyn and Margaret Murnane at JILA, University of Colorado Boulder (USA): the prediction of the temporal emission of the most energetic X-rays generated through the high harmonic generation process [Science 336, 1287 (2012), >1900 citations], and the proposal to generate X-rays from ultraviolet light [Science 350, 1225 (2015), >200 citations]. Furthermore, before the period outlined in this report (2018-2022), the theoretical predictions developed by researchers at the unit were experimentally validated at USAL [for example, Phys. Rev. A 88, 043848 (2013), Opt. Exp. 23, 21497 (2015), Optica 4, 520 (2017)].
These efforts have positioned USAL, particularly LUMES, in a privileged position to conduct research in the emerging field of ultrafast structured light. The focus of the study is on generating high-frequency structured light beams (extreme ultraviolet or even X-rays) with ultra-short durations in the attosecond range. While generating structured laser beams in the visible or infrared range is feasible using optical devices based on light reflection or refraction, shaping such light at higher frequency ranges is challenging, as optical materials progressively become inefficient beyond the ultraviolet. The production of structured light beyond the visible spectrum, therefore, requires a paradigm shift. In 2013, several unit researchers proposed high harmonic generation as a method for the direct production of high-frequency structured radiation, an alternative to imprinting structure on already produced ultraviolet beams. The work [Hernández-García, Phys. Rev. Lett. 111, 083602 (2013), >210 citations] was subsequently experimentally confirmed and is now recognized as a pioneer in the new paradigm for generating high-frequency structured beams.
Noteworthy collaborations published in high-impact journals during the 2018-2022 period, deepening the use of nonlinear optics for the production of structured light beams, include: the generation of isolated circularly polarized attosecond pulses, in collaboration with the group of Prof. Ming-Chang Chen at Tsing Hua Univ. in Taiwan [Nature Photonics 12, 349 (2018), >150 citations]; the discovery of a new property of light beams, self-torque, led by USAL in collaboration with the Kapteyn-Murnane group at JILA, Univ. Colorado Boulder [Science 364 (2019), >200 citations], with broad impact in the public interest (National Geographic, BBC News, El País, El Mundo, etc.); the generation of X-ray vortex beams in collaboration with the Kapteyn-Murnane group at JILA [Nature Photonics 13, 123 (2019), >140 citations] and their understanding in terms of torus-knot angular momentum, in collaboration with ICFO [Phys. Rev. Lett. 122, 203201 (2019), >50 citations]; the generation of vectorial vortex beams in the extreme ultraviolet in collaboration with the group of Prof. Kazamias at Univ. Paris-Saclay [Optica 9, 71 (2022), >30 citations]; or the generation of harmonics with controllable spectral content in X-rays in collaboration with the Kapteyn-Murnane group at JILA [Science Advances 8, (2022), >20 citations]. Researchers from LUMES have collaborated in all these works.
On the theoretical front, contributions have been made to alternative pathways in the field of harmonic generation and electron generation using nanostructures with high conductivity to amplify and modify the laser field at the interaction point with the gas, making electron recombination more effective [PRL 110, 053001 (2013) >100 citations, Rep. Prog. Physics 80, 054401 (2017) >250 citations]. It should be noted that experimentally, this approach is still a challenge as the materials used tend to melt due to the high laser fluence. This problem can be addressed by using ceramic materials, although these materials have very low conductivity, making them inefficient as well. In this scenario, the development of synthetic materials that can withstand high laser fluences while having high conductivities would represent a quantitative leap in the field of high harmonic generation, with implications for structured light, ultrafast magnetism, and more broadly, attophysics.
2. Design of diagnostic tools for structured ultrashort and ultra-intense pulsed lasers
Participating researchers | 4 Principal investigators, 4 senior investigators, 3 PhD students |
Publications related | 15 publications Scientific Reports 8, 3264 (2018), Optics Letters, 44, 2016–2019 (2019), Communications Physics 3, 151 (2020), Optics Letters 45, 3925–3928 (2020), Photon. Res. 10, 922–931 (2022) |
Projects related | National (FIS2017-87970-R, PID2020-119818GB-I00), Regional (SA287P18, SA136-P20, PC-TCUE18-20_050, PC-TCUE18-20_020) |
Patents | US2022407279, US11035737, US11105687, US10651619 |
Theses | One defended, two in progress |
The generation of structured laser pulses with increasingly exotic space-temporal profiles is closely linked to the development of characterization techniques, making this interdisciplinary research line crucial in LUMES. Several researchers in the unit are international references in the development of ultrashort pulse characterization techniques. In addition to designing new characterization techniques in the visible and infrared spectra (such as the STARFISH technique), they are pioneers in the characterization of structured pulses in the extreme ultraviolet. In this regard, the article [Optica 4, 520 (2017), >80 citations] is an international reference for the generation and characterization of vectorial X-ray pulses. This work was developed in collaboration among several unit members, where numerical calculations were performed on the USAL cluster, and experiments were conducted at the Center for Pulsed Lasers (CLPU). Therefore, this is an example of collaboration between researchers and associated infrastructures, now integrated within the LUMES unit.
During the reported period, the work of this research line has focused on the development of spatiotemporal and polarization characterization techniques for structured ultrashort pulses. To achieve this, existing techniques (such as STARFISH) have been adapted to the new spatial characterization requirements, or new techniques (such as BLASHI) have been developed. Notable results have been published in [Communications Physics 3, 151 (2020)], where a comprehensive characterization of ultrafast vectorial pulses is reported, or [Photon. Res. 10 922–931 (2022)], where the characterization of structured ultrafast vortices in time is introduced.
Currently, there are several active synergies within LUMES for the development of advanced diagnostic tools for high-power lasers. These collaborations have not yet resulted in scientific publications, and there is co-supervision of a doctoral thesis focused on the development and use of high-power laser characterization tools.
3. Nonlinear propagation of structured laser beams
Participating researchers | 3 Principal investigators, 4 senior investigators, 4 PhD students |
Publications related | 10 publications Scientific Reports 8, 2256 (2018), Optics Express 26, 6345 (2018), Optics Letters 43, 337 (2018), Optics Letters 47, 5289 (2022), Optics Express 30, 6755 (2022) |
Projects related | National (PID2019-106910GB-I00, PID2020-119818GB-I00, FIS2016-75652-P), Regional (SA287P18) |
Theses | Two defended, three in progress |
In addition to the generation and characterization of structured laser beams, their propagation becomes especially relevant not only for transporting pulses to potential experiments but also for modifying their properties. For over a decade, USAL has been a pioneer in the development of theoretical methods that allow understanding the propagation of ultrafast laser pulses in highly nonlinear media across different spectral regions (far-infrared, infrared, and ultraviolet). In particular, during this period, it has specialized in the study of the nonlinear propagation of light pulses in gas-filled hollow fibers, which can be structured based on the superposition of transverse modes. Noteworthy are the collaborative works with Prof. Helder Crespo at the University of Porto (Portugal), where ultrarapid single-cycle pulses have been achieved [Opt. Lett. 43, 337 (2018), >50 citations; Sci. Rep. 8, 2256 (2018), >40 citations].
Simultaneously, the nonlinear propagation has been studied in antiresonant hollow fibers and multipass cells, in an experimental collaboration with the research group led by Professors Henry Kapteyn and Margaret Murnane at JILA, University of Colorado in Boulder.
Over the past year, in collaboration with the research groups of Professors Matteo Luchinni and Rocío Borrego-Varillas at Politecnico di Milano (Italy) and Professor Travers at Heriot-Watt University, Edinburgh (Scotland), the results on few-cycle pulses propagating through pressure-gradient fibers have been extended [Opt. Exp, 30, 6755 (2022)] towards the generation of high-order harmonics, aiming to produce few-cycle attosecond pulses. Currently, there are two manuscripts submitted for review.
4. Study of the nonlinear interaction of the structured laser beam topology with 2D nanostructured materials
Participating researchers | 4 Principal investigators, 2 senior investigators, 3 PhD students |
Publications related | 11 publications New Journal of Physics, 20, 053033 (2018), Optics Express, 27, 7776–7786 (2019), Optics Express, 29, 2488–2500 (2021), New Journal of Physics, 23, 093011 (2021) |
Projects related | EU (ATTOSTRUCTURA ERC), National (PID2019-106910GB-I00, PID2022-142340NB-I00) Regional (SA287P18) |
Theses | Two defended, one in progress |
The generation of high-order harmonics (HHG) is a strongly nonlinear process known for three decades. It forms the basis for attosecond pulse generation techniques and serves as the most practical solution for generating high-frequency coherent light (extending up to soft X-rays). HHG has undergone two fundamental advances in recent years: (1) the demonstration of the process’s capability to generate structured light, a field in which we were pioneers and extensively explored in research line #1; (2) the replacement of gaseous nonlinear material with nanostructured solid targets. This second aspect has led to the use of HHG not only as a new tool for generating structured light beams but also for exploring the quantum dynamics occurring in solids. The current research line corresponds to our work combining these two scenarios. At USAL, we have developed theoretical models that allowed us to describe HHG in two-dimensional materials, with special attention to graphene. Noteworthy is the work on describing the anisotropy in the nonlinear response of graphene [Optics Express, 27(5), 7776–7786 (2019), >50 citations].
The aspect of the work from the LUMES unit that we consider to have the greatest potential within this research line, which is currently under review in the journal Nature Communications as of the drafting of this report, is a pioneering proposal. In this proposal, it is demonstrated that the topological characteristics of harmonic light enable the identification and resolution of ultrafast dynamics in solids through nonlinear spectroscopy (preprint, https://doi.org/10.21203/rs.3.rs-2161917/v1). These results have prompted the proposal for a theoretical-experimental collaboration between the projects ATTOSTRUCTURA (ERC2019), CHIROTRONICS (ERC2021), and other researchers from the unit. The possibility of implementing the topologically-based nonlinear spectroscopy proposal in the laboratories of USAL is one of the most promising directions for this request from the LUMES unit.
5. Study of ultrafast magnetization driven by laser pulses
Participating researchers | 4 Principal investigators, 4 senior investigators, 2 PhD students |
Publications related | 10 publications ACS Photonics 6, 38–42 (2019), Adv. Sci. 1901876 (2019), Appl. Sci. 10, 1307 (2020), Phys Rev. B 105, 104432 (2022), High Power Laser Sci and Eng 11, e82 |
Projects related | EU (ATTOSTRUCTURA ERC, H2020-MSCAITN-2019-860060), National (PID2020-119818GB-I00, MAT2017-87072-C4-1-P), Regional (SA299P18, SA114P20) |
Theses | Two defended, one in progress |
The future advancement in the development of magnetic memories (MRAM) and other spintronic devices involves developing methods to control the magnetic response on the picosecond (ps) scale. This timescale is significantly shorter than that of existing MRAMs currently in the market, and the most promising control method in this regard is through ultrashort laser pulses. Researchers from the LUMES unit are pioneers in this field, having developed theoretical models that allow studying the dynamics induced by laser pulses, including contributions from the Inverse Faraday Effect and Circular Magnetic Dichroism, as well as thermal effects [V. Raposo et al. Appl. Sci. 10, 1307 (2020)]. This enables the realistic study of these processes and the correct interpretation of experimental results in various ferromagnetic [V. Raposo et al. Appl. Sci. 10, 1307 (2020)], ferrimagnetic [Phys Rev. B 105, 104432 (2022)], and synthetic antiferromagnetic materials [Adv. Sci. 1901876 (2019)]. Additionally, the thermal expansion associated with laser-induced heating in the impact zone propagates in the material as a pulse of elastic stress, which can also affect the magnetic response through magnetoelastic coupling. This effect, often overlooked, can be significant and is currently under study, as it has been observed that mechanical stress gradients can lead to the rapid movement of magnetic textures such as domain walls [M. Fattouhi et al. Phys. Rev. Appl. 18, 044023 (2022)] and skyrmions [M. Fattouhi et al. Phys. Rev. Appl. 16, 044035 (2021)].
On the other hand, structured laser pulses offer a unique possibility in relation to this field of study: the generation of intense and ultrafast magnetic fields, isolated from the electric field [ACS Photonics, 6(1), 38–42 (2019)]. This work has opened a new paradigm in laser-material interaction: the ability to manipulate material properties using the magnetic field, isolated from the electric field. To exploit this potential, a collaboration has been established among various researchers in the unit, framed within the ERC project ATTOSTRUCTURA. In this collaboration, the possibility of coupling spins with the intense magnetic field obtained from an azimuthally polarized vector laser beam has been explored. The first results have already been obtained [High Power Laser Sci and Eng 11, e82 (2023), in press], where the potential to nonlinearly control magnetization using structured laser beams has been discovered. This is one of the most promising research lines within LUMES, in which collaboration among researchers from different disciplines (Optics, Magnetism, Materials Science, Solid-State Physics) is essential.
6. Micro- and nano-structuring of materials with ultrashort pulses, with applications in astrophotonics, biosensing and organ-on-chip
Participating researchers | 1 Principal investigator, 5 senior investigators, 4 PhD students |
Publications related | 52 publications Appl. Surf. Sci. 436, (2018), J. Light. Tech. 37, (2019), Phot. Res. 8, 257 (2020), Prog. Quant. Electron. 72, 100266 (2020), Adv. Photon. Res. 2, (2021), Nature Photonics 17, 59 (2023) |
Projects related | National (PID2020-119818GB-I00, PID2020-119003GB-I00, FIS2017-87970-R), Regional (SA136-P20, SA287P18) |
Theses | Three defended, two in progress |
The unique way in which ultrashort pulses of high-intensity laser light interact with solid targets has opened a thriving field of research in the last decades with countless applications in optical and photonic manufacturing technologies. On one hand, using such pulses makes it possible to create controlled periodic nanostructures on the surface of many materials, thus modifying their surface properties on unprecedented spatial scales. On the other hand, in transparent materials such as glass and optical crystals, the refractive index of the material can be controlled at a micrometer scale, paving the way for the 3D manufacturing of integrated photonic microdevices that, in turn, allow us to control and spatially structure the light propagating through them. For over two decades, researchers from USAL have been working on refining these techniques and their applications.
In recent years, efforts have primarily focused on two lines of work. Regarding the microstructuring of photonic devices, the unit has established itself internationally as a leading group in the integration of waveguides into state-of-the-art transparent crystals. This has led to the development of multifunctional active devices that leverage the excellent properties of these materials. The main application areas for these devices include astrophotonics, where ultra-compact quantum dot spectrometers have been developed in collaboration with ETH Zurich and the European Space Agency [Nature Photonics 17, 59 (2023)], and integrated lasers, achieving ultra-efficient devices [Prog. Quant. Electron. 72, 100266 (2020)]. Current efforts are directed towards incorporating these devices into Organ-on-Chip systems, where they can function as biosensing or control systems, assisted by 2D surface materials.
In the field of surface nanostructuring, in close collaboration for a decade with researchers from the Blas Cabrera Institute of Physical Chemistry and the Institute of Structure of Matter of the CSIC, among others, strategies have been developed to obtain regular surface patterns in the nanometer range in various polymeric and polymer matrix composite materials. This has been achieved using both nanosecond-range pulsed lasers and ultrashort pulses. As a result, processed surfaces are functionalized or enhance pre-existing properties, such as mechanical, chemical, optical, biocompatibility, etc., with applications in biomedicine, sensing, and surface engineering in general [J. Prada-Rodrigo et al. Opt. Laser Technol., 159 (2023); R. Rodriguez-Beltrán., Polymers 14, 5243 (2022)].
7. Fabrication and characterization of electro-optical devices from 2D nanostructured materials
Participating researchers | 2 Principal investigator, 3 senior investigators, 2 PhD students |
Publications related | 13 publications Nature Comm. 9, 659 (2018), NanoLetters 18, 4675 (2018), Nature Nanotechnology 14, 340 (2019), Phys.Rev.Lett. 126, 177401 (2021), ACS Omega, 7, 22626 (2022) |
Projects related | EU (CHIROTRONICS ERC 2021), National (PID2021-128154NA-I00) |
Patents | WO2019219645 |
Theses | Two in progress |
The infinite possibilities of stacking different two-dimensional (2D) crystals in the so-called van der Waals (vdW) heterostructures, as well as structuring the surface of these exotic systems through advanced nanofabrication processes, allow for the design and creation of novel devices with customizable electronic, optical, and magnetic properties on demand.
Researchers from the LUMES unit possess cutting-edge expertise in both the fabrication of high-quality 2D crystals and vdW structures [e.g., Nature Communications 7, 11894 (2016), >400 citations], and in modifying the fundamental properties of these intriguing crystals through nanostructuring processes [e.g., Nature Nanotechnology 14, 340 (2019), >76 citations]. Additionally, this group is a pioneer in the use of such nanostructured 2D crystals to design innovative electro-optical devices and architectures [e.g., Nature Communications 7, 12894 (2016); NanoLetters 18, 4675 (2018)]. These systems operate at room temperature, making them potentially usable in emerging applications such as neuromorphic computing, optical communications, quantum technologies, or precision sensor realization.
On the other hand, through the LUMES excellence unit, multiple synergies among participating researchers have been leveraged for the detailed characterization of van der Waals heterostructures (as well as their corresponding electro-optical devices) through optical measurements. A clear and relevant example is the use of second harmonic spectroscopic measurements in van der Waals structures to determine the coupling that exists among the different 2D crystals composing them and/or the possible presence and eventual utilization of symmetry-breaking in these systems.
8. Nanoscale dynamic properties in novel magnetic materials
Participating researchers | 1 Principal investigator, 6 senior investigators, 2 PhD students |
Publications related | 41 publications Phys. Rev. Lett. 121, (2018), Advanced Science 6, (2019), Applied Physics Letters 115 (2019), Phys. Rev. B 102, (2020), Physical Review Applied 16, (2021), Nature Materials 21, (2022) |
Projects related | EU (H2020-MSCAITN-2019-860060), National (PID2020-117024GB-C41, MAT2017-87072-C4-1-P), Regional (SA299P18, SA114P20) |
Theses | One defended, one in progress |
LUMES researches have extensive experience in the field of computational nanomagnetism. In recent years, their work has focused on materials and heterostructures with asymmetric exchange interaction (Dzyaloshinskii–Moriya),introducing chirality in their properties. They have also explored various materials with antiferromagnetic coupling, such as ferrimagnetic metal alloys, non-collinear antiferromagnetics, and synthetic antiferromagnetics. This research line aims at developing spintronic and magnonic devices with innovative functionalities.
9. Artificial intelligence applied to structured light generation and characterization
Participating researchers | 2 Principal investigators, 2 senior investigators, 1 PhD student |
Publications related | 1 publication Comp Phys Comms 291, 108823 (2023) |
Projects related | EU (ATTOSTRUCTURA ERC), National (PID2022-142340NB-I00) |
Theses | One in progress |
Nonlinear optics and materials science, like most cutting-edge research areas, have not been unaffected by the development of artificial intelligence. In the LUMES environment, we have applied deep learning strategies in two main aspects. Firstly, we have implemented the use of neural networks to enhance our simulation capabilities for laser-material interaction. In a recently published article [Comp Phys Comms 291, 108823 (2023)], we have laid the foundations for integrating artificial intelligence to simulate new scenarios of structured light generation in the X-ray regime. This advancement not only accelerates the simulations we have been conducting to deepen our understanding of laser-material interaction but also allows us to explore new scenarios where traditional simulations may struggle due to high computational loads.
Secondly, we are exploring the use of neural networks for the spatiotemporal characterization of ultrashort pulses. The time required by algorithms used in ultrashort pulse characterization techniques currently limits their use in high-repetition-rate laser systems. Thanks to the implementation of neural networks, the required times can be reduced by several orders of magnitude. Therefore, we are currently investigating the possibility of characterizing structured ultrashort pulses in situ in high-repetition-rate laser systems. This research line has developed in parallel with lines #1 and #3. Although it is in its early stages, due to its interdisciplinary nature and the numerous innovative perspectives it opens up, we believe it will become a relevant research line in the near future of LUMES.
10. Applications of ultrafast optics to materials characterisation
Participating researchers | 3 Principal investigators, 3 senior investigators, 1 PhD student |
Publications related | 2 publications Optics & Laser Technology, 123, 105898. (2020), Optics & Laser Technology, 140, 107018 (2021) |
Projects related | EU (CHIROTRONICS ERC 2021), National (PID2020-119818GB-I00) |
The knowledge gained from the previous research lines can be applied to the characterization of materials’ ultrafast dynamics. Therefore, an emerging line of work in LUMES involves exploring the potential of ultrafast optics in applications for the diagnosis of materials of interest. Initially, the STARFISH technique has been employed to study the behavior of integrated optical systems [Optics & Laser Technology 123, 105898 (2020)]. Expanding on the applications of this technique, it is being adapted for spatial, temporal, and polarimetric sample studies. Ultrafast pulsed rare-earth-doped optical fiber lasers, their stability, and polarization have also been studied [Optics & Laser Technology, 140, 107018 (2021)].
A second line of work aims to exploit the extraordinary potential of ultrafast optics as a tool for time-resolved spectroscopy, a highly versatile technique used in a wide variety of scientific and technological fields to investigate the dynamics of molecular and atomic systems. Its interest lies in its ability to provide information on how systems evolve over time down to the femtosecond (fs) range, with different time scales tailored to the specific characteristics of each study. For this purpose, a first time-resolved fluorescence experimental setup has been implemented using the pulsed laser beam tunable by the laboratory’s optical parametric amplifier (OPA) as the sample’s excitation light. This system can continuously modify the emission spectral region from UV to mid-IR, adapting to the studied sample. The laser beam is directed at a sample holder, and the fluorescence signal is fed into a spectrometer coupled with an intensified iCCD camera with a configurable delay relative to the recorded signal. This setup allows time-resolved fluorescence measurements with a resolution of up to 4 ns. Successful tests of the technique have been carried out. Subsequently, in collaboration with Prof. Mercedes Velázquez’s group at the Faculty of Chemical Sciences of the University of Salamanca, work is underway on the analysis of graphene quantum dot samples, studying their time-resolved spectroscopy to gain a deeper understanding of the properties of such devices. To complete the temporal range, an optical spectroscopic system with femtosecond resolution has been designed and implemented using a pump & probe scheme and proprietary development designs. Initial tests have shown that the system’s resolution is on the order of subpicoseconds. In a second phase, efforts are focused on improving the fluorescence signal collection.